I've been growing onions in my garden for a couple years. Like most of my garden veggies, they're not exactly the typical sort. A few years back I received a large sample of "potato onion" true seed derived from work by
Kelly Winterton. Potato onions are an old-fashioned perennial form of the typical garden onion (
Allium cepa). The "potato" in their name is because they're grown by planting some of the previous year's crop, like as is done with potatoes.
Kelly's introduction into potato onion breeding came from a lucky break, when he planted some of his onions in the fall to see if they could overwinter in the ground at his northerly location. The next season, all of those bulbs flowered prolifically. The important thing he did was to save all those seeds an then try growing them the next year, next to his pre-existing potato onion clones. The high diversity and robust growth of his seedlings caught his attention and started a bit of a movement. (For more details of his work, read through
his site.)
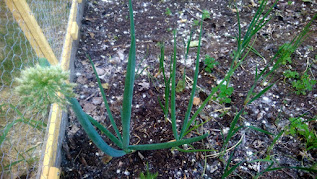 |
Seedling onions, next to seedling Siberian irises. |
I'd read about Kelly's work, so when I had the chance
to get a batch of seeds from one of his lines, I jumped at the chance. My first year working with them, I just tossed a scattering of seeds into a 4"x4" pot and let the seedlings fight amongst themselves for the rest of that year. (I wanted to select for aggressive growers.) At the end of the year, I separated the survivors and planted them in the main garden to overwinter. (I wanted to select for those that were very cold-hardy too.) In the end, I had six plants from that first batch.
Two had lots of luxuriant leafy growth, while the other four seemed to grow a little while and then stall out. I was kinda sad most of the plants didn't seem to do anything, but I left them alone until the first frosty night. As I was pulling up the plants, I got some surprises. All four of the poorly growing plants had grown bulbs, with three of them perfectly formed (though small). The two that grew dramatically produced lots of divisions, but no bulbs.
One of the rapid-growing plants flowered twice over the season, so I was able to collect a next generation of seed. Since the plant didn't bulb up the way I wanted, I found myself with a puzzle. I had no idea if those new seeds would all grow into plants with the same growth habit and no bulbs, or if the nice bulb shape could be produced by some hidden recessive alleles. I really liked the aggressive and early growth shown by this plant, so I didn't want to discard its seeds either.
It took a while of searching before I stumbled on to some useful search queries to get what I was looking for. The first useful paper I found (
Lee et al., 2013) goes into detail examining a set of six genes in
A. cepa that are related to those associated with the control of flowering in the model plant
Arabidopsis thaliana. These "Flowering Locus" genes are transcription factors that regulate how plants develop. The paper has a great deal of interesting information about these genes, but the parts I found most interesting were the experiments showing the interactions between the genes. In this sort of case, I like constructing an
interaction network to help me understand what is going on.
The basic model they came up with encompasses three specific genes (AcFT1, AcFT2, & AcFT4). AcFT2 is induced by sufficient winter cold and then induces flowering. AcFT1 induces bulbing and AcFT4 inhibits AcFT1. Sufficiently long days inhibit AcFT4. All together, we get the network at right.
The two larger inferences we can make from this network are drawn dashed and in color. AcFT4 inhibits bulbing (by inhibiting AcFT1). Sufficient daylight hours stimulates AcFT1 (by inhibiting AcFT4) and thus promote bulbing.
There are a couple more interactions in the biology, so we'll add them. When the flowering pathway is activated in typical onions, the bulbing pathway is suppressed. We'll represent this as a negative influence from AcFT2 to AcFT1, though logically the inhibition could manifest further along the bulbing pathway. The
Lee et al. paper doesn't mention this, but I feel this is justifiable from my experience growing onions through to flowering. An interesting point mentioned in the paper, but not discussed in detail was that AcFT4 over-expression plants showed no senescence of leaves in the fall (in addition to no bulbing), instead growing vegetatively until being stopped by winter.
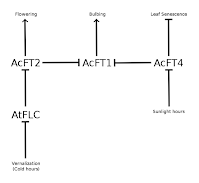 |
Expanded model for onion. |
The
Lee et al. paper describes how vernalization regulates flowering in a few other species. They don't examine in detail how it is happens in onions, but their review of how it is regulated in
Arabidopsis thaliana gives us a good model for how it might work. The variations in the system in different species does highlight how transcription factor networks can easily be rewired to impact development.
The model doesn't clearly indicate the default activities of the genes. AcFT1, AcFT2, AcFT4, and AtFLC are all active by default.
Because AcFT1 and AcFT2 are negatively regulated by AcFT4 and AtFLC, they (and the flowering or bulbing downstream development) are initially inactive.
The authors in
Lee et al. describe how flowering is regulated in a few other model plants for comparison. In short, the same genes are used, but they the comparable interaction network between them has different links. Though the genes are highly conserved, how they work together to drive development is not. The upshot of this is that studies of these genes in other plants is of limited utility to understanding the onions that led me down this path.
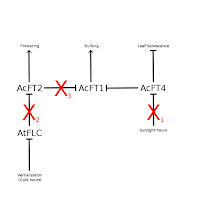 |
Mutations to expanded model for onion. |
Even in onions there is evidence for significant diversity in how this regulatory network is put together. In
Lee et al., the authors hypothesize that leaks may have an overactive FT4 homolog (shown at left as "X
1" on the interaction between daylight hours and AcFT4), resulting in the lack of bulbing and leaf senescence seen in the plant.
Some onions have different vernalization requirements to start flowering. An onion could be entirely resistant to cold as an influence in its blooming, as in "X
2" of this figure. Blooming in these onions would be triggered by other influences not described in my figures, such as plant size or age.
The third mutation I have in this figure ("X
3") breaks the interaction between AcFT2 and AcFT1. This would prevent flowering from interfering with bulb formation and is what seems to be going on in potato onions. When a potato onion blooms, it will form a bulb from a different growth point that is almost as large as if it hadn't bloomed. This is much different than for regular onions, where flowering results in a tiny inedible bulb.
Though there are as of yet no genetic studies illustrating this hypothetical mutation in potato onions, it would be relatively easy to undertake. Potato onions and regular onions are the same species and easily cross (if both are flowering at the same time). Sequencing a large number of F2 progeny would help make a connection between variations in genetic sequence and the phenotype of flowering inhibition of bulbing. It might even be faster to sequentially modify one onion type with mutations to match the FT genes (and surrounding regulatory regions) of the other onion type.
The second technique would at the very least be helpful in validating any findings from the first technique, since what I have indicated as a single interaction could actually involve several other genes. The consequence of this could be that the two FT genes might show now sequence differences at all between the two onion types.
What inspired me to start digging into the biology of bulbing/flowing was the hope that I could make some predictions about the genetics of the potato onions I'm growing.
My robustly growing, but non-bulbing, potato onion seedlings appear to mimic what would be expected if the "X
2" mutation described above was involved. I collected seeds from the best of these plants in hopes that they might contain a recessive bulbing trait that would appear in segregations in the next generation. Unfortunately, I still don't know. The phenotype could be due to either a dominant or recessive mutation. I'll just have to grow out as many of these seeds as I have room for and find out. Answering this question will take another two years, so stay tuned.
I'll also be growing a large number of seeds from the batch I originally received. I wasn't expecting so much diversity to appear in them, so now I'm really interested in what other trait combinations will turn up.
References: